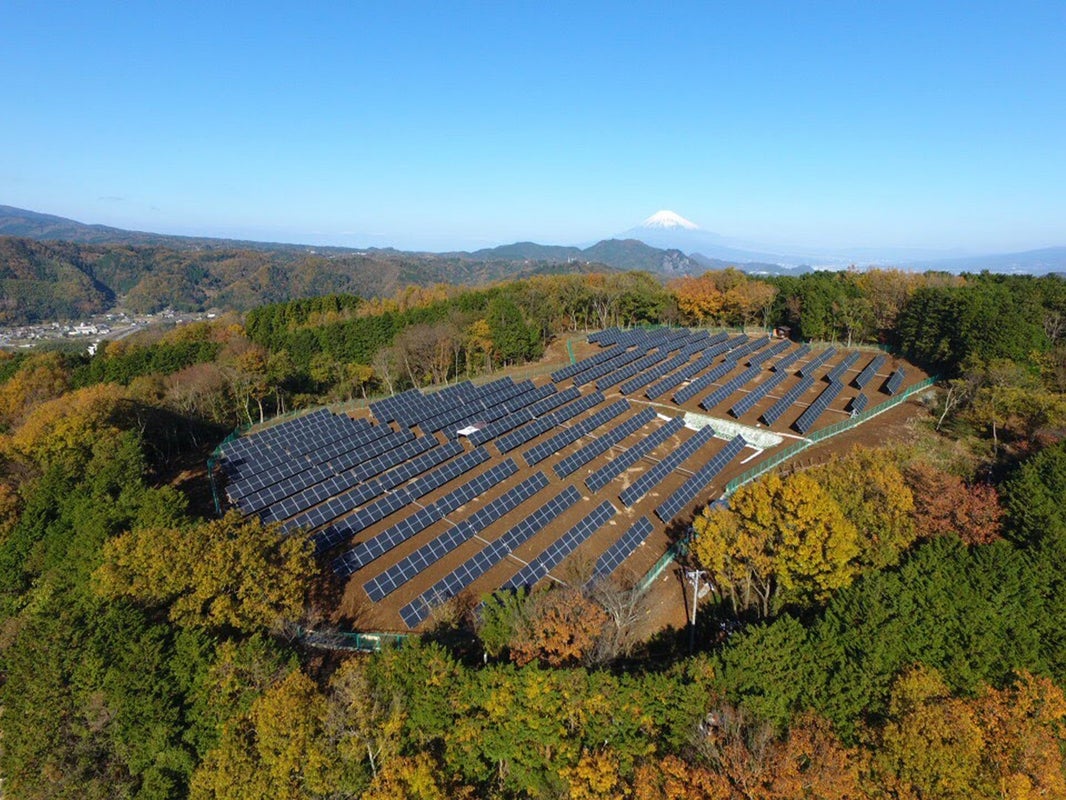
1. Principles of Solar Panels (Photovoltaic Effect)
Solar panels work based on the photovoltaic effect, which is the process by which sunlight is converted into electricity. Here's a breakdown of the principles:
a. Photons and the Semiconductor Material
- Sunlight consists of energy packets called photons, which are particles of light.
- Solar panels are made of semiconductor materials (typically silicon) that absorb these photons.
- When photons hit the semiconductor material in the solar cells, they impart their energy to electrons in the material, knocking them free from their atoms.
- This results in the formation of free electrons (negatively charged) and holes (positively charged).
b. P-N Junction and Electric Field
- Solar cells consist of two layers of semiconductor material: the p-type (positive) and n-type (negative) silicon layers.
- At the junction of these two layers, a built-in electric field forms, which acts as a diode, preventing the free electrons and holes from recombining.
- The electric field drives the free electrons toward the n-type layer and the holes toward the p-type layer.
c. Flow of Electrons and Creation of Electric Current
- The electrons flow through an external circuit (the load) from the n-type to the p-type layer, creating an electric current.
- This direct current (DC) electricity can be used directly or converted to alternating current (AC) using an inverter for general consumption (homes, industries, etc.).
2. Efficiency Issues in Solar Panels
The efficiency of a solar panel refers to how effectively it converts sunlight into usable electricity. Conversion efficiency is a critical factor for both residential and commercial solar installations because it affects how much space and how many panels are required to meet energy needs. Various factors influence the efficiency of solar panels:
a. Material Properties and Energy Conversion Efficiency
- The most common semiconductor material used in solar panels is silicon, which is effective in converting sunlight into electricity. However, the efficiency of silicon-based panels is limited by several factors, including:
- Bandgap: The bandgap of silicon (around 1.1 eV) determines the energy required to free electrons. This is a key factor in the panel’s ability to absorb and convert light into electricity.
- Spectral Range: Silicon absorbs light only within a certain range of the electromagnetic spectrum, meaning some sunlight (especially ultraviolet and infrared light) is not fully utilized.
- Multijunction Cells: To overcome the spectral limitation of single-junction solar cells, multijunction solar cells are developed, where multiple layers of semiconductors absorb different parts of the light spectrum. These are more efficient but are also more expensive.
b. Conversion Losses
Solar panels are subject to various conversion losses at different stages of energy transformation:
- Reflection Losses: When sunlight strikes the surface of the solar panel, a portion of the light is reflected back into the atmosphere instead of being absorbed. This can be minimized with anti-reflective coatings.
- Thermal Losses: As solar panels absorb sunlight, they also heat up. Higher temperatures can reduce the efficiency of the panels, as heat causes the semiconductor material to lose energy. This is why solar panels perform better in cooler climates with plenty of sunlight.
- Recombination Losses: When free electrons and holes recombine before being collected by the electric field, energy is lost as heat. Advanced panel designs, such as PERC (Passivated Emitter and Rear Contact) and bifacial technologies, help reduce recombination.
c. Temperature Effects
- Temperature Coefficient: Solar panels are less efficient at higher temperatures. The typical temperature coefficient for silicon-based panels is around -0.4% to -0.5% per degree Celsius above 25°C (77°F).
- As panels heat up under intense sunlight, their efficiency decreases because the increased thermal energy interferes with the motion of electrons in the semiconductor material.
d. Shading and Angle of Incidence
- Shading: Even partial shading on a solar panel (from trees, buildings, or other obstructions) can lead to significant efficiency losses because it disrupts the flow of electrons. This is especially problematic in series-wired solar panel systems.
- Angle of Incidence: The angle at which sunlight strikes the panel also affects its efficiency. Fixed panels often need to be oriented optimally to maximize energy capture, depending on the latitude, time of year, and sun path.
e. Manufacturing Defects and Panel Degradation
- Manufacturing Quality: Imperfections in the manufacturing process can affect the electrical performance of the solar cells, leading to lower efficiency. Companies invest in high-precision fabrication processes to minimize defects.
- Degradation Over Time: Over the lifetime of a solar panel (typically 25-30 years), its efficiency decreases slightly due to wear and tear, exposure to the elements, and degradation of the semiconductor material. Most panels degrade at a rate of around 0.5% per year, but the impact can vary depending on the quality of the materials used.
3. Key Issues Affecting Solar Panel Efficiency Conversion
While modern solar panels have achieved significant progress in terms of efficiency, several key issues continue to affect their overall performance and conversion efficiency:
a. Theoretical Efficiency Limits
- Solar cells made from silicon have an upper theoretical efficiency limit of about 29%, known as the Shockley-Queisser limit. This limit is due to the fact that silicon can only absorb a narrow band of light energy, and the rest of the energy is either lost to heat or cannot be effectively converted.
- Multijunction cells can exceed this limit by using different materials to capture a broader range of wavelengths, but these cells are much more expensive.
b. Light Absorption Efficiency
- A major limitation for many solar panels is their ability to absorb and utilize sunlight. Thin-film technologies or concentrated solar power (CSP) systems aim to address these limitations, but they also come with higher costs or the need for more space.
c. Cost vs. Efficiency Trade-off
- Achieving higher efficiency often involves using advanced technologies (e.g., bifacial cells, PERC, HJT - Heterojunction technology), which tend to be more expensive. For residential systems, it may not always be cost-effective to opt for high-efficiency panels, particularly in regions where space is plentiful, and lower-efficiency panels can still provide the required energy output.
4. Solutions and Innovations to Improve Efficiency
Several innovations are currently underway to address the efficiency issues of solar panels:
a. Passivated Emitter and Rear Contact (PERC) Cells
- PERC cells have an additional passivation layer at the back of the solar cell, which reduces recombination losses, increases light absorption, and boosts overall efficiency by up to 1-2%.
b. Bifacial Solar Panels
- Bifacial panels capture sunlight on both the front and the rear sides, increasing energy production. The rear side can absorb reflected light from the ground, which can increase efficiency by up to 30% in certain conditions.
c. Heterojunction Solar Cells (HJT)
- HJT technology combines silicon with thin layers of other materials, such as amorphous silicon, to enhance efficiency. These cells are expected to reach efficiency levels above 25%, while maintaining low-temperature coefficients.
d. Tracking Systems
- Solar tracking systems allow solar panels to follow the sun's path throughout the day, maximizing exposure and increasing efficiency by up to 30% over fixed panels.
Add comment
Comments